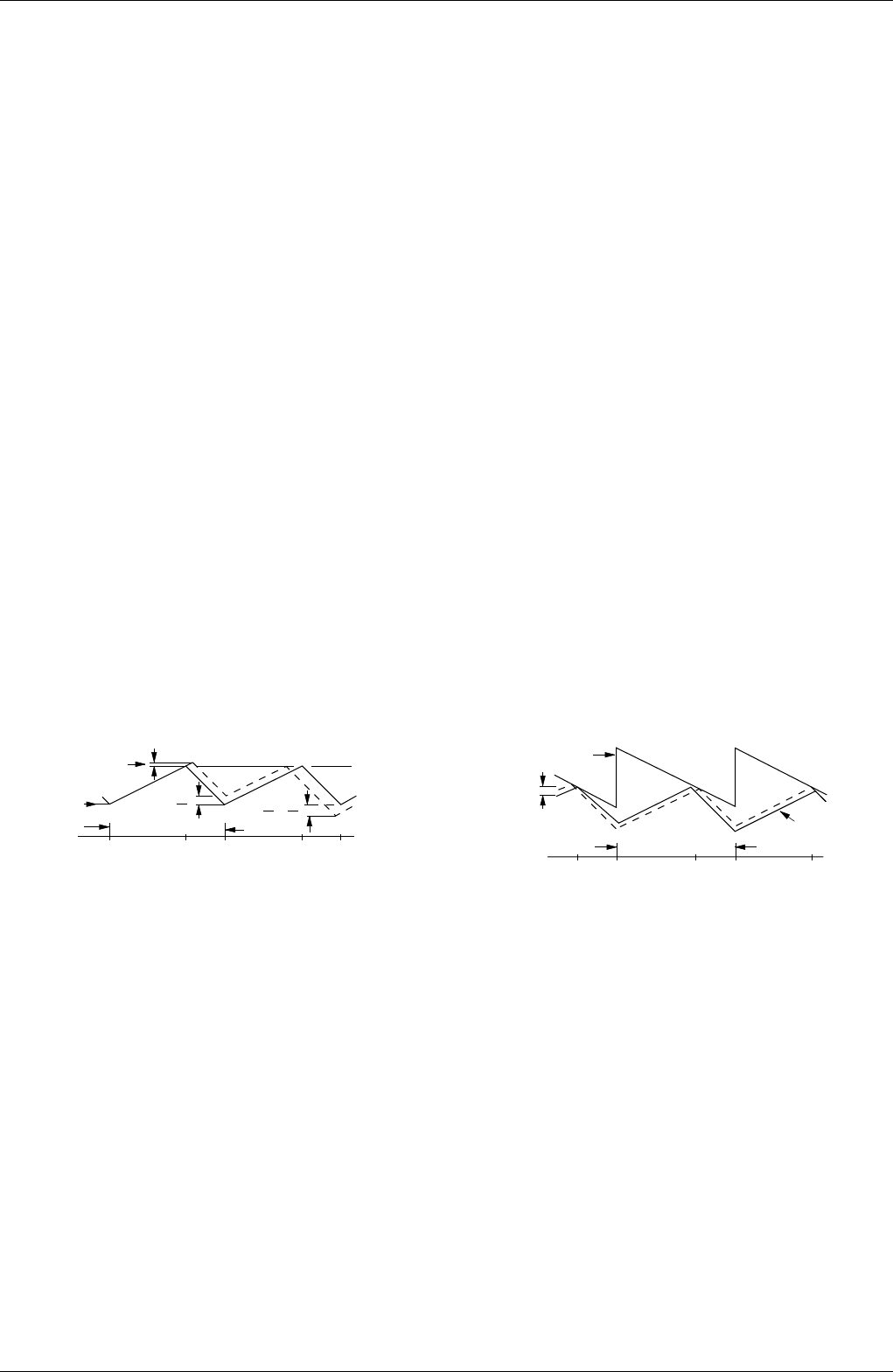
CUC 7350
Schaltungsbeschreibung / Circuit Description
GRUNDIG Service 2 - 1
Circuit Description
1. Power Supply
1.1 Basic Circuit
Current mode converters can exhibit subharmonic oscillations when
operating at a duty cycle greater than 50% with continuous inductor
current. This instability is independent of the regulators closed loop
characteristics and is caused by the simultaneous operating condi-
tions of fixed frequency and peak current detecting.
Figure 1 shows the phenomenon graphically. At t
0
switch conduction
begins, causing the inductor current to rise at a slope of m
1
.This slope
is a function of the input voltage divided by the inductance. At t
1
, the
Current Sense Input reaches the threshold established by the control
voltage. This causes the switch to turn off and the current to decay at
a slope of m
2
, until the next oscillator cycle. The unstable condition can
be shown if a pertubation is added to the control voltage, resulting in
a small ∆l (dashed line). With a fixed oscillator period, the current decay
time is reduced, and the minimum current at switch turn-on (t
2
) is
increased by ∆l + ∆l m
2
/m
1
. The minimum current at the next cycle (t
3
)
decreases to (∆l + ∆l m
2
/m
1
) (m
2
/m
1
). This pertubation is multiplied by
m
2
/m
1
on each succeeding cycle, alternately increasing and decreas-
ing the inductor current at switch turn-on. Several oscillator cycles may
be required before the inductor current reaches zero causing the
process to commence again. If m
2
/m
1
is greater than 1, the converter
will be unstable. Figure 1 shows that by adding an artificial ramp that
is synchronized with the PWM clock to the control voltage, the ∆l
pertubation will decrease to zero on succeeding cycles. This compen-
sating ramp (m
3
) must have a slope equal to or slightly greater than
m
2
/2 for stability. With m
2
/2 slope compensation, the average inductor
current follows the control voltage yielding true current mode opera-
tion. The compensating ramp can be derived from the oscillator and
added to either the Voltage Feedback or Current Sense inputs (Figure 2).
Fig. 2
1.2 Normal / Controlled Operation
For the power supply of this TV receiver a blocking oscillator-type
converter power supply with a switching frequency of 50kHz approxi-
mately is used (at normal operation and a mains voltage of 230V).
The drain contact of the power transistor T60020 is connected via the
primary winding 1/3 of the blocking oscillator-type transformer TR60020
to the rectified mains voltage, D60011…D60014. At a mains voltage of
230V the voltage level present at the charging electrolytic capacitor
C60029 is approx. +320V.
The IC60030 is responsible for driving, controlling and monitoring the
MOS power transistor T60020. The supply for the control-IC is 12V and
is present on Pin 7. As soon as the switch-on threshold is reached on
Pin 7 via the resistor R60017 and the capacitor C60031, the IC feeds
out a positive start pulse (1µs) of 10V
p
at Pin 6. After start-up of the IC,
the supply voltage is obtained via the diode D60031 from the winding
5/7 of the transformer. During the conducting phase of the transistor,
energy is stored in the transformer and this is transferred into the
secondary winding when the transistor is switched off. The IC60030
controls by the period during which the transistor T60020 is switched
on, the transfer of energy at Pin 6 so that the secondary voltages are
stable and are largely not affected by variations of the mains supply,
mains frequency and the load.
The power transistor T60020 is driven by a pulse-width modulator
which is triggered by an oscillator integrated in the IC. The frequency
of the oscillator is determined by the components C60041 and R60042.
For stabilisation, the feedback voltage which is rectified by D60047 is
compared in IC60030 with the 5V reference voltage provided at
Schaltungsbeschreibung
1. Netzteil
1.1 Prinzipschaltung
Stromrichter können subharmonische Schwingungen aufweisen, wenn
sie mit einem Arbeitstakt > 50% bei kontinuierlichem Induktionsstrom
betrieben werden. Diese Instabilität ist unabhängig von den Eigen-
schaften geschlossener Reglerkreise und wird durch die gleichzeitige
Messung der Festfrequenz und des Spitzenstroms verursacht.
In Fig. 1 ist diese Erscheinung graphisch dargestellt. An t
0
beginnt der
Einschaltvorgang und damit steigt der Induktionsstrom mit einer
Steigung m
1
an. Dieser Anstieg ist eine Funktion der Eingangs-
spannung im Verhältnis zur Induktanz. An t
1
ist die maximale Strom-
stärke erreicht, die von der Steuerspannung festgelegt ist. Dadurch
wird die Sperrphase eingeleitet und der Strom fällt in einer Kurve m
2
ab
bis zum nächsten Schwingungsvorgang. Die Instabilität läßt sich
zeigen, indem man ein Störsignal zur Steuerspannung addiert. Daraus
ergibt sich die kleine Stromänderung ∆I (gestrichelte Linie). Bei einer
festen Schwingungsdauer verkürzt sich die Sperrphase und die Mindest-
stromstärke in der Leitphase (t
2
) erhöht sich um ∆I + ∆I m
2
/m
1
. Die
Mindeststromstärke beim nächsten Zyklus (t
3
) fällt auf (∆l + ∆l m
2
/m
1
)
(m
2
/m
1
) ab. Diese Störgröße multipliziert sich mit m
2
/m
1
bei jedem
folgenden Zyklus, so daß der Induktionsstrom beim Umschalten der
Polarität abwechselnd steigt und fällt. Bis der Induktionsstrom Null
erreicht, sind mehrere Schwingungszyklen notwendig. Anschließend
beginnt der Vorgang von neuem. Ist m
2
/m
1
größer als 1, wird der
Stromrichter instabil. Addiert man zur Steuerspannung eine künstliche
Sägezahnspannung, die mit dem Pulsbreitenmodulations-Takt syn-
chronisiert wird, wie in Abbildung 1 dargestellt, verringert sich die
Störgröße ∆I in den nachfolgenden Zyklen und wird Null. Damit eine
Stabilität erzielt werden kann, muß die Steilheit dieser Korrektur-
spannung gleich oder etwas größer als m
2
/2 sein. Bei einer Korrektur-
spannung von m
2
/2 richtet sich der durchschnittliche Induktionsstrom
nach der Steuerspannung, so daß sich eine echte Stromregelung
ergibt. Die Korrekturspannung wird aus dem Oszillator abgeleitet und
entweder dem Spannungsrückkopplungs- oder dem Strommeßein-
gang zugeführt (Fig. 2).
Fig. 1
1.2 Normalbetrieb / Regelbetrieb
Zur Stromversorgung des Gerätes wird ein Sperrwandlernetzteil mit
einer Schaltfrequenz von ca. 50kHz verwendet (bei Normalbetrieb und
einer Netzspannung von 230V).
Der Drainanschluß des Leistungstransistors T60020 liegt über der
Primärwicklung 1/3 des Sperrwandlertrafos TR60020 an der gleich-
gerichteten Netzspannung, D60011…D60014. Am Ladeelko C60029
steht bei 230V Netzspannung ca. +320V.
Die Ansteuerung sowie die Regel- und Überwachungsfunktionen des
MOS-Leistungstransistors T60020 übernimmt der IC60030. Die Versor-
gungsspannung des Regel-ICs (Pin 7) liegt bei 12V. Nach dem
Erreichen der Einschaltschwelle an Pin 7 über den Widerstand R60017
und den Kondensator C60031 gibt der IC an Pin 6 einen positiven
Start-Impuls (1µs) von 10V
s
ab. Nach dem Anlauf des ICs wird die
Versorgungsspannung über die Diode D60031 aus der Wicklung 5/7
des Wandlertrafos gewonnen. Während der Leitphase des Transistors
wird Energie im Übertrager gespeichert und in der Sperrphase über die
Sekundärwicklung abgegeben. Der IC60030 regelt an Pin 6 über das
Tastverhältnis des Transistors T60020 so nach, daß die Sekundär-
spannungen weitgehend unabhängig von Netzspannung, Netzfrequenz
und Last stabil bleiben.
Den Leistungstransistor T60020 steuert ein Impulsbreitenmodulator
an, der von einem im IC integrierten Oszillator getaktet wird. Die
Frequenz bestimmen die Bauteile C60041 und R60042. Zur Stabilisie-
rung vergleicht der IC60030 die über D60047 gleichgerichtete
Rückkopplungsspannung mit der Referenzspannung von 5V an
IC60030-(8). Sinkt die Rückkopplungsspannung durch größere Last
∆l + ∆l
m2
m
m
2
1
∆l + ∆l
m
m
2
1
m
m
2
1
())(
m1
Inductor
Current
Control Voltage
∆l
t
0
t
1
t
2
t
3
Oscillator Period
(A)
t
45
tt
6
∆l
m1
m2
m3
Inductor
Current
Oscillator Period
Control Voltage
(B)